“Thus there is not merely one world, one earth, one sun, but as many worlds as we see bright lights around us…”
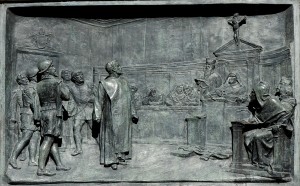
Written in 1584 by Giordano Bruno, these words mark the first serious consideration that there might be other solar systems besides our own: that the stars we see are like our own Sun and that they may have Earths of their own. Bruno was an early heliocentrist, an adherent to the theory that the Sun, not the Earth, was at the center of the universe. Early Greek philosophers had suggested that the Sun was simply a star that is much closer to us than all other stars, but until Copernicus put forward evidence that the Earth revolves around the Sun, no one had any reason to think that there may be planets orbiting other stars. Maybe Bruno got caught up by the Copernican spirit: that our place in the universe was nothing special. Maybe he just wanted to rankle Catholic orthodoxy. But in the third dialogue of his treatise On the Infinite Universe and Worlds, he reasoned that if our place wasn’t in the privileged central position in the universe, maybe there was no center of the universe, and every star had planets around it. He even went so far as to hypothesize that these planets hosted life forms with rational beings who would no doubt agree with all the conclusions he was promulgating. For these writings (among others), Giordano Bruno was convicted of heresy and burned at the stake in 1600.
Nowadays, astronomers tell us that there are at least 854 confirmed extrasolar planets, planets orbiting stars other than our Sun, and there are thousands of “candidates,” possible extrasolar planet discoveries that are awaiting independent confirmation. In the space of 400 years, we’ve gone from executing people who even hinted at the existence of planets outside our solar system to proclaiming that there are hundreds or even thousands of planets within only a few hundred light years of us. That’s a big jump. So how can we now prove the idea that Giordano Bruno died for four centuries ago?
Take a picture of it
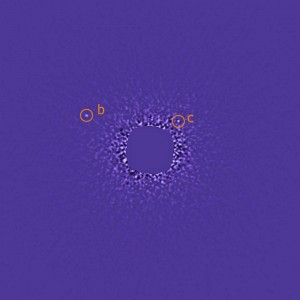
The most straightforward way to prove that planets exist around other stars is also one of the most difficult. As the host star is thousands or even millions of times brighter than the planets surrounding it, its light almost completely washes out the light of the planets. There have been significant advances in subtracting a star’s light from an image in an attempt to eliminate this problem, but to date, only a handful of planets have had their pictures taken. One of the first systems detected in this manner is shown at left. The strange blank spot and fuzzy image are artifacts of the software algorithm used to subtract the light from the parent star (HR 8799) out of the image. The image was taken in 2008, which suggests that the technique of directly taking images of alien worlds is still in its infancy. Later analyses of the HR 8799 system would produce pictures showing that the star has at least two additional planets orbiting it, for a total of at least four planets.
Watch an eclipse of it
The Kepler space telescope has been lighting up science blogs and newswires ever since its launch in early 2009. The NASA-operated spacecraft, along with its counterpart COROT, operated by the European Space Agency, is tasked with measuring the light from over 100,000 stars with extreme precision, recording the brightness of these stars to about 20 parts per million. If something passes between the star and the telescope, the brightness of the star dips. If the same dips are spotted repeatedly and on a regular schedule, this indicates that the “something” between the star and the telescope is a planet, because it is in orbit around the star and is thus eclipsing the star once per orbit. By measuring how much the brightness of the star dips, we can determine how large the planet is. By measuring the duration of the dip and the frequency with which it happens, we can determine the orbit of the planet. This method of planet detection is called the transit method, and it’s probably easier to describe with a picture:
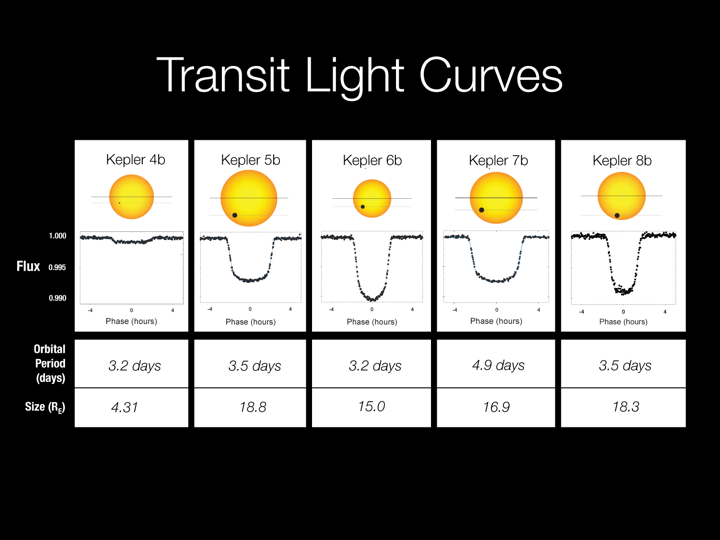
The data from Kepler and COROT is striking in its simplicity. In fact, these dips are so characteristically easy to spot (and the data gathered so far is so overwhelmingly plentiful) that scientists have begun to ask for the public’s help in trying to spot these needles in the haystack of data that they’ve collected. If you’ve got a few minutes to kill, you can maybe discover the next alien planet at www.planethunters.org, but I warn you, it’s really easy to kill way more than a few minutes over there.
The drawback to the transit method is that a lot of stars have a natural variability to their brightness, so the method generates a lot of false positives. As such, this method is usually used to screen for possible extrasolar planet “candidates,” and their existence is usually confirmed by some other method.
Watch for the star to wobble
When most people think about gravity, they imagine an apple falling to Earth, or maybe the planets flying around the sun, or moons flying around planets. In general, the word “gravity” invokes a mental image of a large object (like the Earth) pulling a small object (like an apple) toward it. But in reality, gravity is a two-way street. Remember Newton’s third law: Every action has an equal and opposite reaction. So the apple is pulling just as hard on the Earth as the Earth is on the apple. It’s just that the apple doesn’t have nearly as much mass to pull with, so the Earth moves a much smaller distance toward the apple than the apple moves toward the Earth. But, according to Newton, the Earth still moves toward that apple. In fact, a physicist would tell you that both the Earth and the apple are falling toward an imaginary point called the center of mass. How can you tell what the center of mass of the Earth-apple system is? Well, the mass of your average apple is about 100 grams, or 0.1 kilograms. The mass of the Earth is about 6,000,000,000,000,000,000,000,000 kilograms. You can divide one weight by the other to figure out how far, relatively speaking, the Earth and the apple move. So for every meter the apple moves toward the earth, the Earth will move toward the apple about 0.000000000000000000000017 millimeters, or roughly a billionth of the width of an atomic nucleus. This is an unimaginably short distance, so we are pretty much correct in our intuition that an apple falls to Earth and not the other way around.
But what happens if something bigger (much, much bigger) is falling to Earth? What if, for example, the Moon were to suddenly fall to Earth? How far would the Earth move toward the Moon? We can answer this question the same way we answered the one above. The mass of the Moon is very roughly about 1/100th the mass of the Earth. This means for every 100 miles the Moon fell toward the Earth, the Earth would fall toward the Moon about 1 mile. Since the Moon is currently about 250,000 miles away, the Earth would fall toward the moon about 2500 miles. This is definitely a noticeable distance.
What does all of this have to do with detecting planets around other stars? As I said before, we typically think of gravity acting in one direction: a star’s gravity makes planets orbit around it. But if we let gravity act in both directions, we find out that the orbiting planet actually tugs on the star a little bit, too, causing the star to wobble back and forth as the planet goes around it. From the way that the star wobbles, we can deduce how big the planet in orbit around it is and how far away from the star it is.
Ok, you say, so you just watch the star to see if it wobbles, right? Actually, not quite. It turns out to be extremely difficult to directly see tiny wobbles in a star that’s trillions of miles away. So how do we know it’s wobbling if we can’t see it wobble? We use the Doppler effect. You might have heard of the Doppler effect with respect to weather radar or speeding tickets, and you might even know that the Doppler effect is the reason that a police car’s siren sounds like it’s dropping in pitch as it passes us on the freeway. But what does the Doppler effect have to do with stars wobbling? How does the Doppler effect even work, for that matter? Take a look at the picture below:
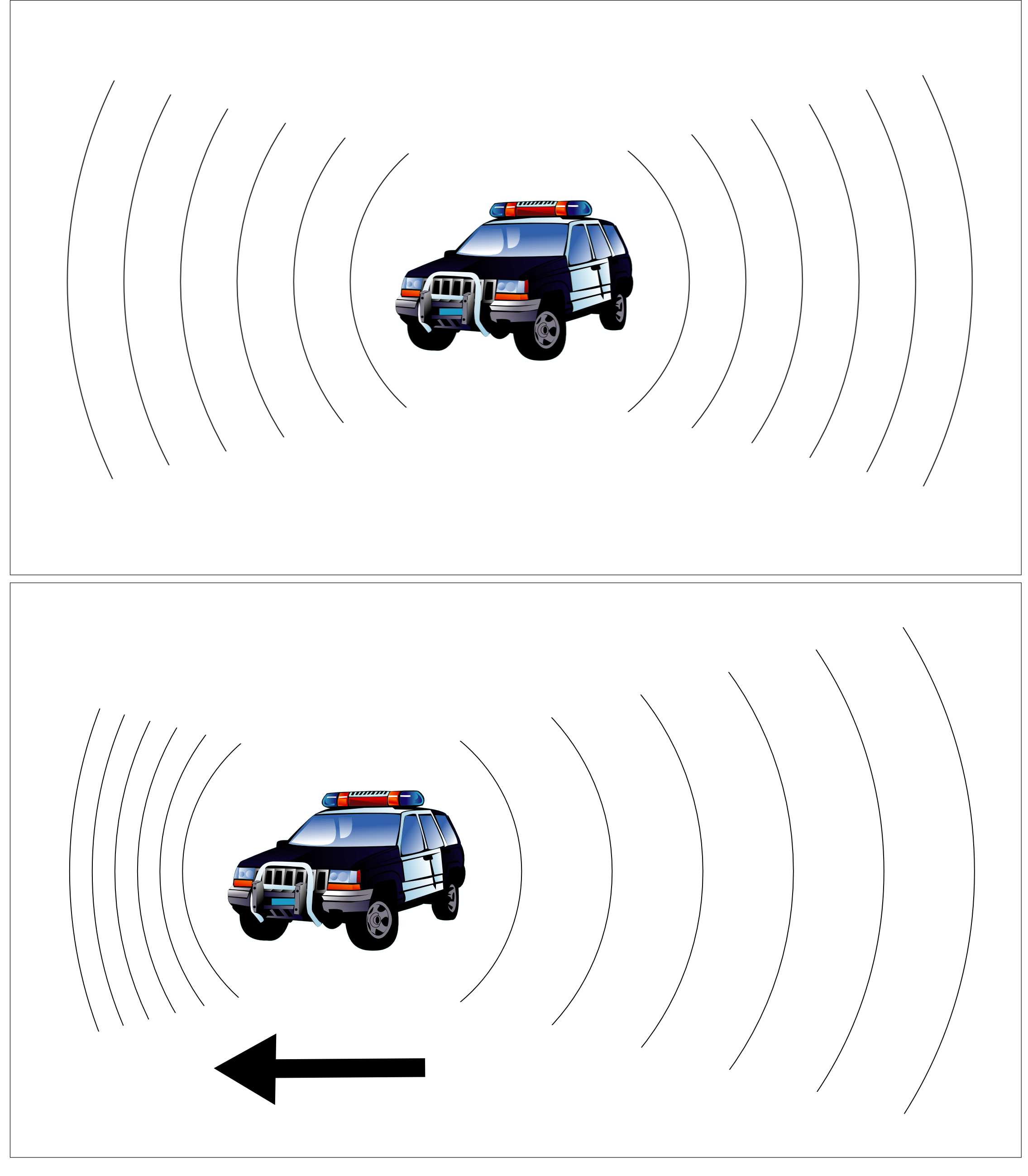
Bottom: When the police car starts moving, the sound waves ahead of the car pile up on each other, causing the siren’s pitch to rise. The sound waves behind the cruiser are stretched out, so the siren’s pitch falls.
When a police car is not moving, its siren doesn’t change in pitch. That’s because the sound waves that come from the siren all come marching out the same distance from each other in every direction. This is pretty easy to see in the top picture. This distance between waves, or wavelength, is what determines the pitch that our ears hear. The longer the distance, the lower the pitch. But now, if the police cruiser starts to drive to the left with its siren on, the waves that are moving to the left come out closer to one another than the waves that are moving to the right. This is because, as the siren is emitting each new sound wave, it’s moving toward the left-hand waves that have already been emitted, and at the same time it is moving away from the right-hand waves. So we hear the close-together waves on the left as a higher pitch, whereas we hear the far-apart waves on the right as a lower pitch. This is why a police siren or a train whistle sounds like it’s dropping in pitch as it passes.
But sound waves aren’t the only waves that undergo the Doppler effect. In fact, any type of wave, be they water, sound, or even light waves, will undergo the Doppler effect. It’s the Doppler effect observed in light waves that astronomers look for when they want to see a star wobble. Just like the passing police car, as a star moves toward us, the “pitch” of the light goes up, so to speak. In reality, what this means is that the light from the star gets ever so slightly more blue, because the shorter wavelength means higher energy, which happens to correspond to blue light. And as the star moves away from us, its “pitch” goes down. In other words, the wavelengths of light from the star appear longer, so the star appears more red. This is what scientists often mean when they refer to blueshift and redshift. They’re referring to the fact that the Doppler effect causes the color of light that they see to change ever so slightly.
Because the Doppler effect merely requires looking at the color of the light coming from the host star, and not at tiny variations in the intensity of light or tiny pinpricks of light from the planets themselves, the star wobble technique is the oldest way of finding planets orbiting around other stars. Astronomers break up a star’s light into its constituent colors, just like you can do with a prism and a flashlight, and they look for changes in these colors. If they see a star’s light becoming blue, then red, then blue, then red in a stable recurring pattern, they can deduce that the star is wobbling. Based on the mass of the star, and the regularity with which the star is wobbling, they can figure out the size of the object that’s tugging on the star. If they find that the object is in the right mass range, they’ll usually declare that the star has a “planet candidate.” Generally what that means is that other astronomers should probably take a look at the star, and if they see the same effect as the first astronomers, only then can they say with any confidence that the star has a planet orbiting it.
How many planets are out there?
We’ve looked at the three most common planet-hunting tools in astronomers’ arsenals: direct imaging, transit dimming, and Doppler shifting. There are a few other ways that astronomers use, but these three are the main ones. So how many planets are there? Well, there have been enough discoveries and corroborations at this point that it looks like, on average, there is at least 1 planet per star in the galaxy. That means that, in our galaxy alone, there are probably at least 100,000,000,000 planets. And that’s not to mention other galaxies, which we have no reason to believe will be too terribly different from our own. Maybe somewhere out there, some warm planet has given rise to life of its own. And maybe out there, that life has evolved to the point where it has begun practicing science. And just maybe, it’s discovering for the first time just how many planets are actually out there. And maybe, just maybe, it’s wondering whether some warm planet has given rise to life of its own….
References
- G. Bruno, “On the Infinite Universe and Worlds,” in Giordano Bruno: His Life and Thought by Dorothea Waley Singer. New York, Henry Schuman: 1950.
- A wealth of information about the transit method and the Kepler space mission can be found at kepler.nasa.gov
- The paper estimating the number of planets in the galaxy is:
- “One or more bound planets per Milky Way star from microlensing observations” Nature 481, 167–169, and can be found here.
3 replies on “How do we know that there are planets orbiting other stars?”
If you are interested in doing some Citizen Science on looking for Exoplanets check out Planethunters.org
Turns out the human brain is still the best pattern recognition computer out there so they ask for people to identify transit light curves like Keith described. If enough people identify the same star as having transients, they point a big ol’ telescope at it and confirm it. They are about to publish their first find PH-1 in Science! If you are the first to document the transient you even get to be included on the paper! In any case, it’s a fun way to spend 5-10 minutes. Plus its a Yale University program!
Hey there, You’ve done an excellent job. I will certainly
digg it and personally suggest to my friends. I am sure they will be benefited from this web site.
It seems that proof of other planets hinges on one important principle, the principle of ‘maybe’……..